Metals in Sediments
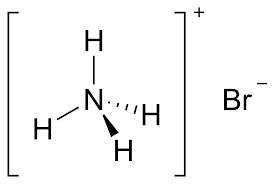
Bromine, resulting from the charged Zn–Br2 battery, is dissolved in a nonaqueous phase electrolyte contained in the aqueous electrolyte. The bromine can be stored as a complex species with quaternary ammonium cations contained in the electrolyte and forming tetramethyl ammonium bromide-bromine, N-ethyl-N-methyl-morpholinium bromide-bromine, trioctyl methyl ammonium chloride-bromine, and polymeric salts such as polydiallyldimethyl ammonium bromide. The quaternary ammonium salts form a high-density emulsion containing bromine and this is separated from the aqueous electrolyte, containing lower amounts of bromine, as a dense liquid. This phase generally contains both a miscible organic solvent and the quaternary ammonium polybromide salt. These methods are a convenient way to contain and store bromine, allow low rates of self-discharge, reduce the reactivity and vapor pressure of bromine, improve safety, and diminish bromine traveling toward the negative electrode where it can be reduced at the zinc electrode. A porous or ionic separator between the positive and the negative electrode also partially prevents the transport of bromine to the zinc electrolyte compartment. The bromine emulsion circulates through the cell with the aqueous electrolyte and is separated by gravity in the storage tank. As a result, the electrolyte contains an aqueous phase with diluted bromine and a phase rich in polybromine. Most of the bromine-rich phase sits at the bottom of a second internal reservoir, contained in a larger reservoir for this electrolyte. In some systems, the positive electrolyte containing bromine enters the cell at the top and leaves from the bottom manifold. This avoids the accumulation of the bromine-rich phase in the bottom of the cell.
One of the problems with the Zn–Br2 battery is the rate of self-discharge caused by the migration of bromine into the zinc electrode compartment. Concentrations of up to 2 mol dm?3 of bromine can be dissolved in an aqueous electrolyte during charge and an ion-exchange membrane is required to reduce bromine transport. If an ion-exchange membrane is not available, the common strategy is to use the quaternary ammonium bromide salt in the bromine electrolyte to reduce bromine migration. The salt absorbs most of the bromine generated during the charge cycle to leave an aqueous solution of approximately 0.1 mol dm?3 concentration and offers the possibility of using a microporous separator. An alternative option when a microporous separator is used is to add propionitrile (PN) as a solvent for the bromine generated during charge. Propionitrile is immiscible in water and helps to decrease the self-discharge rate. An example of a Zn–Br2 cell based on PN–water electrolytes consists of a flat titanium negative electrode (zinc) of 16 cm2 surface area. For the positive electrode (bromine), two different materials were tested: spot-welded platinum gauze to titanium plate and a plastic-bonded carbon electrode. A microporous plastic 0.6 mm in thickness separated the two electrodes forming the negative and positive electrolyte compartments. The gap between each electrode and the separator was 0.7 mm. The electrolyte volumetric flow rate in both compartments was 2 cm3 s?1 and the cell was charged and discharged at a constant current of 25 mA cm?2 at 298 K. It was found that the PN electrolyte containing bromine leaked to the zinc electrode compartment and reduced the overall energy efficiency of the cell to around 38%. However, high energy efficiencies of 66% could be obtained when the plastic separator was interleaved between two filter paper discs. The combination of PN, a separator, and a quaternary ammonium bromide salt increased the overall energy efficiency to 93%. The disadvantages of using PN are its low conductivity, in comparison with the aqueous phase together with its toxicity and flammability. Some advantages of PN addition to the electrolyte include a lower viscosity and a low-energy pumping requirement.
Porous silica NPs are formed when active ingredients are adsorbed inside the NPs without forming any linkage. Surfactant forms the basic skeleton on which silica oxides are deposited and condensed. The removal of the surfactants results in the synthesis of these NPs. These NPs are nontoxic and biocompatible with large surface area and tunable pore size and volume. They offer a variety of surface functionalizations, which can be used for controlled release of pesticides against plant pathogens. Mesoporous silica NPs and hollow silica NPs are two major forms of inorganic porous NPs. The functionalized mesoporous silica NPs are absorbed in the insect cuticular membrane lipids, damaging their protective waxy layer and causing dehydration reported that loading of avermectin pesticide in the inner core of mesoporous silica NPs resulted in their protection against photodegradation and slow release. In this study, mesoporous silica NPs were prepared in a step-by-step process. Calcium carbonate was dissolved in distilled water and surfactant (hexadecyltrimethyl ammonium bromide) was added. After heating at 353 K, sodium silicate was added with continuous stirring. Two hours later, the suspension was filtered and vacuum dried at 373 K. The calcium carbonate shell was removed after calcination by HCl treatment. The NPs were collected after filtration and vacuum dried. Avermectin was then loaded into the prepared mesoporous silica NPs by supercritical carbon dioxide technology. Acetone was added to the mixture and kept in high pressure adsorption apparatus, which after vacuum drying formed mesoporous silica NP-loaded avermectin. The UV shielding properties of the avermectin-loaded porous hollow silica NPs were evaluated along with the effect of temperature and pH of the medium.
Because of the entrapment of the avermectin, photostability of the pesticide increased. However, UV shielding properties of entrapped avermectin declined with increased intensity of UV, pH, and temperature. Similarly, Cao reported successful loading of pyraclostrobin into mesoporous silica NPs, which resulted in sustained release of the pesticide. The capping of the mesoporous silica NPs with water-soluble chitosan resulted in increased pesticide loading efficiency and faster release rate than bare mesoporous silica NPs. The fungicidal activity of the encapsulated pesticide against Phomopsis asparagi was studied and it was reported that half doses of pyraclostrobin-loaded capped mesoporous silica NPs possessed the same fungicidal activity.
- Art
- Causes
- Crafts
- Dance
- Drinks
- Film
- Fitness
- Food
- Games
- Gardening
- Health
- Home
- Literature
- Music
- Networking
- Other
- Party
- Religion
- Shopping
- Sports
- Theater
- Wellness
- IT, Cloud, Software and Technology